Abstract
Keywords: attention, visual orienting, alerting, EEG, ADHD
The current pilot study was conducted for a proposed intervention examining the use of video gaming to improve visual orienting among a normative sample of individuals without attention deficits. The authors hypothesize that a daily regimen of attention-related activities might facilitate improved visual orienting and vigilance. An experimental 6-week intervention including intensive computer game playing was administered to a normative sample of eight undergraduates. Electroencephalogram (EEG) recordings taken during a visual orienting test (VOT) before and after the gaming intervention indicated an increase in alpha activity during resting conditions and a decrease during alerting conditions. The current results suggest that intensive video gaming may ultimately remediate attention-related deficits among individuals with Attention Deficit/Hyperactive Disorder .
Pilot Study: The Effect of Video Gaming On Attention-Related EEG Activity
A pilot study was conducted to test a proposed experimental intervention that has been designed to facilitate improvement with specific attention-related skills. The pilot study was conducted with a normative, sample of undergraduates, all of whom were free of any prior diagnoses of Attention Deficit Hyperactivity Disorder (ADHD). This intervention involved a six-week regimen of playing arcade-style computer games with the goal of fostering improvements in the participants’ ability rapidly shift their attention (visual orienting). The results of the current pilot study were intended to be used in the development of a larger intervention study to examine the feasibility of using a similar intervention to help individuals with ADHD improve their attention-related skills. The current data were also used for the purposes of power analyses to estimate target sample sizes for the proposed larger study.
It has been argued that visual orienting (the ability to shift attention from one location to another) is problematic for some individuals with ADHD (Fisher, 1998; Voeller & Heilman, 1988). For example, Collings and Kwasman (2006) found that children meeting the diagnostic criteria for ADHD-Inattention type (ADHD-IA) responded significantly more slowly to stimuli appearing in the periphery than did their non-ADHD counterparts. It was hypothesized that these children have a disproportionately difficult time becoming or remaining alert for impending targets, especially those that appear in the periphery (Collings & Kwasman, 2006; Collings & McClaren, under review). This hypothesis is supported by research that has focused on a baseline resting condition. When compared to non-ADHD individuals, persons with ADHD often have insufficient alerting states, illustrated by deficiencies in mean alpha activity during Electroencephalogram (EEG) recordings (Clarke, Barry, McCarthy, Selikowitz, & Brown, 2002). It seems plausible that this cortical hypoarousal might hinder ADHD individuals’ ability to respond quickly to non-attended stimuli (Clarke, Barry, & McCarthy, 2003).
This hypothesis compliments Posner and Petersen’s (1990; Posner & Raichle, 1994) model of attention that identifies a neural system related to becoming alert for impending stimuli. When cued by a stimulus, this vigilance system quiets brain activity, allowing the individual to react more rapidly to subsequent stimuli. Several authors have suggested that some individuals with ADHD may have deficits in these vigilance systems, preventing these individuals from quieting their brain activity, consequentially impairing their ability to become and remain alert (Posner & Raichle, 1994; Schaughency & Hynd, 1989). Such deficits might cause these individuals to respond slowly to events and stimuli or to miss them entirely (Posner & Raichle, 1994).
It is possible, however, that such vigilance-related deficits may be fulfilled through new advances in the area of neural plasticity. Recent research in the area of stroke rehabilitation suggests that these patients are able to reorganize neural pathways in response to intensive behavioral interventions (Holloway, 2003). This therapeutic approach, referred to as Constraint-Induced Movement Therapy, consists of rigorous regimens of physical exercises involving impaired motor skills. Provided that the regimen is sufficiently intense (typically daily), significant improvement in motor function frequently occurs within weeks. Neural imaging studies have demonstrated that these improvements in motor function are correlated with the reorganization of neural systems in non-damaged brain areas (Taub & Uswatte, 2003). We hypothesize that a similar therapeutic approach involving an intensive regimen of appropriate attention tasks might enable individuals to develop more efficient vigilance processes and a more typical state of alertness.
In the current preliminary study, we piloted an experimental intervention in which a normative, non-ADHD sample of undergraduates participated in a six-week regimen of daily attention tasks. These tasks consisted of playing video games that required rapid responses to stimuli appearing in the periphery. EEG recordings were conducted during week one (pretest) and week seven (posttest). In keeping with Clarke et al. (2002), we hypothesized that mean alpha activity levels would significantly increase in the experimental group from pre- to post-intervention during the baseline (non-vigilant) condition compared to the control group who received no intervention. Based on Clark et al. (2002), we predicted that the intervention would result in improved vigilance processes, reflected in significant decreases in mean alpha activity levels during alert conditions from pre- to post-intervention.
Method
Participants
We recruited seven undergraduate students as volunteers from psychology classes for the experimental group in this study. However, because we lost the posttest EEG recordings for one of these participants due to a corrupted data file, the data from that student was omitted from the current analyses. The remaining six participants (two males and four females, mean age = 19.7 years) had a mean IQ = 106.14. One participant was left-handed. Although two participants scored high on inattention and hyperactivity, no participants reported a prior diagnosis of ADHD or learning disability. The participants received class credit for their participation. Five students (all female) were recruited as a control group (mean age = 20.00, mean IQ = 104.00). Because two control participants dropped out after the pre-testing session, and because we lost data for the posttest EEG measurements for a third student, our control sample included only the remaining two control participants. Consequently, the data from this group were not included in the primary analyses, and were analyzed with a separate series of post hoc analyses.
Materials
Paper and pencil measurements included the Current Symptom ADHD Scale (CSS), a 4-point Likert-type scale questionnaire with items derived directly from the ADHD symptom list in the DSM-IV. We employed the Wonderlick Personnel Test (WPT) and Health History Questionnaire (HHQ) to measure intelligence and prior health problems. We used the Edinborough Handedness Inventory (EHI), a 4-point Likert-type scale questionnaire, to determine the handedness of each participant. Finally, we utilized a Visual Acuity Screening (Snellen Chart) to screen for vision problems that might have interfered with the procedure.
We used a Visual Orienting Task (VOT, Posner 1980) as a reference task in conjunction with EEG observations measured by a Mindset MS-1000 (Nolan Computer Systems, CO) 16-channel topographic neuromapping instrument. For this we employed a standard EEG protocol with 16 electrodes positioned based on the 10-20 electrode system integrated into a Lycra stretchable cap with ear lobe referents. The VOT was administered on a Pentium IV Laptop with 512mb RAM and a 15” UXGA Display. A standard keyboard was used for responses, and an adjustable chinrest was used to minimize head movements1.
Experimental Intervention
For the intervention protocol, participants played a n assortment of 24 computer games requiring responses to moving objects appearing peripherally on the computer screen. Participants played games for 90-minute sessions (with breaks) Monday through Friday for each of six weeks. We used a game set representing several genre including; (a) classic arcade games (“Millipede”, “Asteroids”, “Breakout”, etc.), (b) racing games (“Speedboat Racer”), and (c) sports games (“FIFA Soccer”). Participants kept daily video game diaries to record the number of hours each participant spent playing computer games outside of the laboratory sessions.
Design and Procedure
During the first week, participants completed several pre-test assessments consisting of several paper and pencil measurements, a visual screening, and a computer-based VOT with simultaneous EEG. The VOT measured participant reaction time when shifting attention. Each trial began with the individual fixating on a “+” in the middle of the screen. After 900ms, a cue (highlighted box) appeared on either side of the fixation point, presenting a box in that location. After either 150ms, 750ms, or 1250ms, a target stimuli was presented either in the cued location (valid condition, 30% of trials), or to the opposite visual field (invalid condition, 30% of trials). As soon the target appeared, the participant responded with a keystroke. Also included were a neutral cue condition, wherein both visual fields were cued (30% of trials) and a no cue condition (10% of trials) for a total of 96 trials.
EEG samples, recorded at 1024 observations per second, provided data on alpha wave (8-13Hz) activity for the following electrode locations: Fp1, Fp2, Fz, F3, F4, F5, F7, F8, Cz, C3, C4, Pz, P3, P4, T3, T4, T5, and T6. After collecting the EEG data, we compiled the data sets and determined average alpha activity amplitudes by isolating 500ms prior to the cue (baseline) and 600ms after the cue (cued state). We examined the stream and excluded the artifacts. EEG data samples were constructed by combining both the valid and invalid cues since both should make the participant equally alert. We omitted neutral cues from the current analyses because they contained dual-cues and may have alerted the participant in a different manner than single cue conditions. Similarly, no cue trials were omitted because they contained no alerting cue to prompt shifting attention. We constructed four regional alpha wave amplitude averages by collapsing electrodes (1) Fp1, F3, and F7 (left anterior), (2) Fp2, F4, and F8 (right anterior), (3) P3 and T5 (left posterior), and (4) P4 and T6 (right posterior).
During the six-week period of in-lab gaming intervention following the pretest sessions, participants recorded personal gaming time in their diaries. At the end of the gaming intervention (week seven), we subjected them to a second EEG/VOT assessment in the same manner as the pretests. Although control subjects did not visit the lab to play computer games during weeks one to six, they did complete the daily gaming diaries via email.
Results
We computed a series of 3-way repeated measures ANOVAs to ascertain the effect on mean alpha amplitude, the dependent variable (DV), for three independent variables (IVs): (a) intervention (pre and post), (b) hemisphere (left and right), and (c) location (anterior and posterior). These analyses were performed for baseline and post-cued conditions.
Not surprisingly, given the small sample sizes, there were no significant main effects for the intervention, hemisphere, or location on average alpha amplitude during the baseline (non-cued) condition. Nor were there significant interactions, although the effect size for the intervention by location interaction appeared to be moderately large, F(1,5) = 1.36, p = .296, η = .46. The intervention appeared to increase the mean alpha activity during resting states, although this increase was much less pronounced in the left hemisphere anterior locations (Figure 1). Similar analyses for the cued condition indicated no significant main effects or interactions. However, the main effect for intervention, F(1,5) = 2.28, p = .191, η = .56, as well as the intervention by location, F(1,5) = 3.46, p = .122, η = .64, and intervention by hemisphere by location interactions, F(1,5) = 1.05, p = .352, η = .42 were relatively large. As expected, during alert states the overall alpha activity declined. However, these declines appear to have varied across locations and to a lesser extent across hemispheres (Figure 2).
Although the control sample was small (n=2), we conducted a series of post hoc analyses to rule out the possibility that the aforementioned effects may have resulted from an order effect, rather than the intervention. Although not significant, the two-way intervention X group (experimental vs. control) interaction effect on mean alpha amplitude during the baseline condition was large, F(1,6) = 2.13, p = .194, η = .51. We observed no pretest/posttest increase in alpha activity in the anterior regions with the control group. Similar analyses in the posterior regions and in the cued conditions revealed no significant or large differences between the experimental and control groups.
Discussion
Although significant results were not obtained when testing for mean alpha activity pre- and post-intervention for both the baseline and vigilant conditions, the large effect sizes reflected a trend toward increases in mean alpha activity in the experimental group for the baseline condition. This trend was more prominent in the anterior than the posterior location. We also observed a trend toward decreases in alpha activity for the vigilant condition, which was again more prominent in the anterior than posterior location with the experimental group. The large (albeit non-significant) effect for the contrast between the mean alpha activity for the experimental and control groups would suggest that we simply had too little power to detect the intervention’s effect, not surprisingly given the small sample used in the current pilot study. The current findings would suggest, however, that the intervention effect on baseline alpha activity would be localized to frontal areas.
Clearly, the results of the current pilot study are limited by the small, non-clinical sample and by the lack of an adequate control group. Although we found indications of potential changes in alpha activity with non-ADHD individuals, our findings may or may not generalize to persons with ADHD. However, we are hopeful that the large effects noted in the experimental group would predict replication in future studies. If so, such results may provide a foundation for devising interventions for individuals with ADHD by facilitating improvements with specific attention-related skills (i.e., visual orienting and vigilance).
Specifically, the post-intervention increase in alpha activity during the baseline condition might be good news for individuals with ADHD who have been found to exhibit cortical hypoarousal (Clarke et al., 2002). Following a similar intervention, the brains of individuals with ADHD may develop a more effective alerting state through reorganization of neural systems (Holloway, 2003; Taub & Uswatte, 2003), allowing them to shift their attention more appropriately. Similarly, we are hopeful that this intervention would improve the vigilance deficits in individuals with ADHD. Following such an intervention with individuals who are unable to effectively remain alert for impending stimuli, we are hopeful that cue stimuli might trigger a decrease in the alpha activity during alerting states, enhancing the processes of detecting and responding to stimuli.
The current pilot data are being used to develop a protocol and power analyses for a larger study to examine the efficacy of the using such an intervention to remediate visual orienting and vigilance deficits among individuals with ADHD. We are cautiously optimistic that a rigorous battery of attention-related tasks, provided by computer games similar to those used in the current pilot would eventually offer an effective alternative or complement to pharmacological interventions for this disorder.
References
Clarke, A. R., Barry, R. J., & McCarthy, R. (2003). Effects of stimulant medications on children with attention-deficit/hyperactivity disorder and excessive beta activity in their EEG. Clinical Neurophysiology, 114, 1729-1737.
Clarke, A.R., Barry, R.J., McCarthy, R., Selikowitz, M., & Brown, C.R. (2002). EEG evidence for a new conceptualization of attention deficit hyperactive disorder. Clinical Neurophysiology, 113, 1036-1044.
Collings, R. D., & Kwasman, A. (2006). Visual orienting deficits among ADHD-Inattentive Type children. Individual Differences Research, 4, 111-122.
Collings, R. D., & McClarren, S. (2006). Spatial and Alerting Effects in the VOT: Beyond the Spotlight. Manuscript submitted for publication.
Fisher, B. C. (1998). Evaluation of Attention Deficit Disorder. Attention Deficit Disorder Misdiagnosis: Approaching ADD from a brain-behavior/neuropsychological perspective for assessment and treatment. Boca Raton, Fl: CRC Press, pp. 271-293.
Holloway, M. (2003). The Mutable Brain. Scientific American, 79-85.
Jenks, M. J., LeTarte, K., & Collings, R. D. (2006, March). Pilot study: The effects of video gaming on visual orienting and vigilance. Poster session presented at the annual conference of the Eastern Psychological Association, Baltimore, MD.
Posner, M. I. (1980). Orienting of attention. Quarterly Journal of Experimental Psychology, 32, 3-25.
Posner, M. I., & Petersen, S. E. (1990). The attention system of the human brain. Annual Review of Neuroscience, 13, 25-42.
Posner, M. I., & Raichle, M. E. (1994). Networks of attention. Images of Mind, New York: Scientific American Library, pp. 153-180.
Schaughency, E. A., & Hynd, G. W. (1989). Attentional control systems and the Attention Deficit Disorders (ADD). Learning & Individual Differences, 1, 423-449.
Taub, E., & Uswatte, G. (2003). Constraint-induced movement therapy: bridging from the primate laboratory to the stroke rehabilitation laboratory. Journal of Rehabilitation Medicine, 35, 34-40.
Voeller, K. K., & Heilman, K. M. (1988). Attention deficit disorder in children: A neglect syndrome? Neurology, 38, 806-808.
Authors’ Note
Funding for the current study was provided by a Faculty Research Grant through the Research Foundation at the State University of New York College at Cortland (Raymond D. Collings, Principal Investigator). The Authors would like to acknowledge the following student research assistants for their help with the project: Suzanne Karp, Victoria Puzo, Kaitlyn Murphy, Melissa Jenks, Ivano Iaia, Troy Lichten, Kelly LeTarte, Anthony Nelson, Diella Giaconia, Andrew Drago, Peter Scialdone, Miranda Hendrickson, Adrianne Fuller, Merlann Pena, Emily Raynor, and Elizabeth Sinclair. We would also like to thank Drs. Leslie Eaton and Jeffrey Swartwood for their assistance with the EEG data collection. Portions of the data presented here were presented at the 2006 annual conference for the Eastern Psychological Association in Baltimore.
Correspondence should be addressed to the third author, Dr. Raymond D. Collings, Department of Psychology, State University of New York College at Cortland, P.O. Box 2000, Cortland, NY 13045. He may be contacted at 607-753-2046 or [email protected].
Figures
Figure 1. Mean alpha level for baseline condition. (back)
Note: Mean alpha activity during 500ms following onset of fixation during valid and invalid cue conditions. Fp1, F3, and F7 (left anterior), Fp2, F4, and F8 (right anterior), P3 and T5 (left posterior), and P4 and T6 (right posterior).
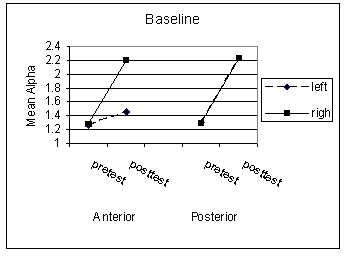
Figure 2. Mean alpha level for cued condition. (back)
Note: Mean alpha activity during 600ms following onset of cue during valid and invalid cue conditions. Fp1, F3, and F7 (left anterior), Fp2, F4, and F8 (right anterior), P3 and T5 (left posterior), and P4 and T6 (right posterior).
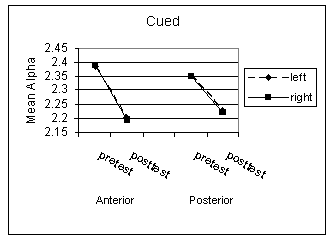
1Data from the VOT were not analyzed as part of the current study, but are reported in a separate study (Jenks, LeTarte, & Collings, 2006). (back)